TRPL imaging enables researchers to map the photoluminescence lifetime across a sample with micrometer-scale spatial resolution. It combines pulsed laser excitation, confocal or widefield microscopy, and precise time-correlated single photon counting (TCSPC) to reveal localized differences in charge carrier dynamics, defects, and material inhomogeneities.
To achieve this, each detected photon is not only time-stamped relative to the excitation pulse, but also assigned to a specific pixel in the image. This creates detailed lifetime maps that go beyond conventional intensity-based fluorescence imaging.
A typical TRPL imaging setup consists of:
PicoQuant offers a range of modular platforms tailored for TRPL imaging, from compact widefield systems to fully modular confocal microscopes – all supporting picosecond to millisecond time resolution. Explore below to find the system that best suits your materials research needs.
TRPL Imaging Microscope Platforms
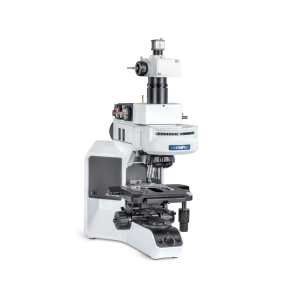
Compact upright widefield microscope for time-resolved photoluminescence (TRPL) measurements.
Offers fast and reliable acquisition of steady-state and time-resolved luminescence from UV to NIR, with spatial resolution down to 2 μm – ideal for material screening, quality control, and comparative TRPL studies.
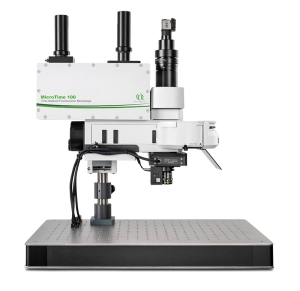
Compact and flexible confocal microscope for time-resolved photoluminescence (TRPL) applications. Offers picosecond to microsecond lifetime resolution, broad spectral coverage, and upgrade options such as SNSPD integration, temperature control, and charge carrier diffusion modules – best suited for materials research from solar cells to single emitters.
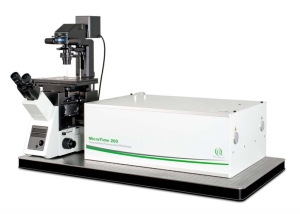
High-end confocal microscope system for advanced time-resolved photoluminescence (TRPL) studies—down to the single-photon level. Enables true confocal TRPL imaging with picosecond to millisecond lifetime resolution, dual-channel detection, and full modular upgradeability – especially effective for demanding material science research involving quantum dots, 2D materials, and photonic nanostructures.
Questions? Contact us >
Carrier diffusion in a GaAsP quantum well system
Carrier diffusion observed in a GaAsP quantum well system. The central spot of the image was illuminated with a 440 nm pulsed diode laser at 40 MHz and the emission was filtered with a 735/50 nm bandpass filter. Only the detection volume was scanned over the image. The false color code in the different images of the ROI indicates that the average lifetime increases for larger radii from the point of illumination. This is shown with a complete lifetime mapping in (a) and individual ROI of increasing distance from the excitation in (b), (c) and (d). A closer look at the temporal behavior of the TRPL as shown in (e) reveals that the maximum in the transient photoluminescence shifts to later times in the transient emission for increasing distance from the point of illumination. This is the result of the longer time scales for the migration of charge carriers from the center of the image into the selected region of interest before emitting a photon from recombination. While the rise times vary, the primary decay component remains constant at around 1.1 ns. Only the center of the image shows a different behaviour, where the decay is slightly shorter at roughly 1 ns since diffusion from the point of excitation acts as an additional depleting component.
Set-up:
Sample courtesy of Andrea Knigge, Ferdinand-Braun-Institut, Leibniz-Institut für Höchstfrequenztechnik, Germany.
CdTe-polycrystalline wafer surface
A CdTe-polycrystalline wafer surface was scanned on a confocal microscope before and after thermal activation with a chloride compound. The respective intensity images (a) and (b) as well as the lifetime images (d) and (e) before and after treatment show a significant increase in intensity and photoluminescence lifetime after activation. The statistical distribution of the intensities (c) and lifetimes (f) over the full image are given before (blue) and after (green) activation. With only the 3 ms/pixel measurement time, a distinctive change in the average lifetime can be determined as well as significant variations of the lifetime over different regions of the CdTe structure.
Set-up:
Sample courtesy of Hannes Hempel and Christian Kraft, Institut für Festkörperphysik, Friedrich-Schiller-Universität Jena, Germany
References:
[1] Buschmann, V. et al, Journal of Applied Spectroscopy, Vol.080, p.449-457 (2013)
[2] Kraft, C. et al, Journal of Applied Physics, Vol.113, 124510 (2013)
Latest 10 publications related to TRPL
The following list is an extract of 10 recent publications from our bibliography that either bear reference or are releated to this application and our products in some way. Do you miss your publication? If yes, we will be happy to include it in our bibliography. Please send an e-mail to info@picoquant.com containing the appropriate citation. Thank you very much in advance for your kind co-operation.